In partnership with the editors for the Fundamentals of Maternal Anatomy and Physiology (Peate and Leader, 2024), and Fundamentals of Maternal Pathophysiology (Leader and Peate, 2024), this series provides an evidence-based summary of the cardiac, respiratory and endocrine systems. This third paper focuses on the endocrine system.
The endocrine system was previously considered a discrete system. However, it works with the nervous and immune systems, co-ordinating and regulating the body's physiology in response to changes inside and outside the body. A diverse pathophysiology of the maternal endocrine system exists, which includes diabetes mellitus, hyperemesis gravidarum and disorders of the adrenal, thyroid and pituitary glands, as well as the hypothalamic–pituitary–adrenal axis. It is important for midwives to be aware of the role of hormones and endocrine function during pregnancy, birth and postnatally, to ensure women receive appropriate care.
The endocrine system
Many endocrine responses are initiated by neural triggers and the hormones produced (chemical messengers) often act as neurotransmitters too. However, whereas the nervous system responds rapidly, endocrine responses can range from seconds to days, providing slower but more precise adjustments to body functions. A comparison can be seen in Table 1.
Nervous system | Endocrine system | |
---|---|---|
Speed of action | Seconds | Minutes to hours (or days) |
Duration of action | Seconds to minutes | Minutes to days |
Method of transmitting messages | Electrical | Chemical |
Transport method | Neurones | Hormones |
Hormones produced by the endocrine system are responsible for maintaining homeostasis, a stable internal environment in the body. This includes regulating tissues, organs and the composition of body fluids, sustaining bodily functions, such as the menstrual cycle, triggering survival mechanisms in response to changes in the external environment and activating or inhibiting the immune system. The endocrine system is intrinsic to metabolism and growth, but also behaviour and sexual differentiation and development. It plays a key role in all aspects of female reproduction, from the release of the mature ovum, successful implantation and the maintenance of the embryo in early pregnancy, through the development of the fetus, placenta and uterus, to the physiology of labour and birth, lactation and early relationships.
The human endocrine system involves the production of around 50 hormones from several glands and cells throughout the body. Endocrine means ‘secreted into the bloodstream’, in contrast to exocrine, which means ‘secreted into a duct or opening’, for example sweat, tears or saliva (Knight, 2021). Endocrine glands are very vascular, making it easy for hormones to diffuse into the bloodstream. They are distinct structures, such as the pituitary, thyroid or adrenal glands. However, hormones are also produced in endocrine cells within organs and tissues, such as the gastrointestinal tract, pancreas or ovaries. Some hormones are produced in several places; for example, melatonin, a hormone associated with sleep and biorhythms, is produced in the pineal gland but also in a wide range of organs and tissues in the body.
During pregnancy, the production of many hormones increases. Temporary structures, such as the placenta and decidua (endometrium of uterus), are responsible for significant hormone production and the fetus can produce some hormones too. Many endocrine changes in pregnancy are the result of hormonal signals from the fetal–placental unit, critical in directing the initiation, maintenance and adaptations of pregnancy, fetal development and the birth process. Immediately after birth and expulsion of the placenta, many hormone levels drop or change to facilitate lactation. The endocrine system gradually returns to its pre-pregnancy state, although levels of some hormones may take up to 12 weeks to do so. Table 2 summarises a selection of the major hormones relevant to human reproduction.
Name | Primarily secreted from | Action | |
---|---|---|---|
Oxytocin | Posterior pituitary gland | Uterine contraction, lactation (milk ejection), social behaviour, bonding | |
Prolactin | Anterior pituitary gland | Lactation (milk production) | |
Growth hormone | Anterior pituitary gland | Cell growth, energy for fetus | |
Melatonin | Pineal gland, various organs | Regulates sleep and biorhythms | |
Thyroxine, triiodothyronine | Thyroid gland | Regulates metabolism | |
Thymopoietin, thymosin, thymulin | Thymus gland | Stimulates T-cell production (immune response) | |
Gonadocorticoids: sex hormones | Oestrogens, eg oestriol Androgens, eg testosterone Progesterone | Adrenal gland cortex, ovaries, placenta and fetus | Fertility, male/female characteristics, libido Oestrogen stimulates uterine growth and activity, blood supply, preparation of breasts for lactation Progesterone acts on smooth muscle, inhibits uterine activity in pregnancy |
Relaxin | Ovaries and decidua | Stimulates uterine and cardiovascular changes, relaxes pelvic ligaments | |
Human chorionic gonadotrophin | Trophoblast cells and placenta | Stimulates thyroid, maintains corpus luteum, linked to nausea and vomiting | |
Human placental lactogen | Trophoblast cells and placenta | Metabolic modification to supply energy to fetus |
Secretion, transportation and action of hormones
Hormones are transported around the body in the blood, either in the plasma (water-soluble hormones) or bound to transport proteins (fat-soluble hormones). Some act locally on adjacent cells, known as paracrine signalling. Hormones bind to target cells, altering the function of these cells. Some act on specific target cells, ie those with specific receptors in their cell membranes, but others affect a wide range cells, for example thyroxine. The action of hormones depends on how they are secreted and the response of their target cells. Secretion and regulation is influenced by many factors; the nervous system (neural stimulation), the availability of binding proteins, chemicals or nutrients in the blood (humoral), by environmental changes or through the influence of other releasing or inhibiting hormones (hormonal).
Regulation and excretion
Hormone release and levels are regulated through the processes of positive and negative feedback. Positive feedback speeds up processes, such as the production of prolactin from the anterior pituitary gland during lactation, optimised through early and regular, responsive breastfeeding. Prolactin directs lactocyte cells in the breast to produce milk. Suckling by the infant stimulates prolactin production and thus milk production increases. If suckling decreases or stops, prolactin production will be reduced and subsequently the amount of milk produced declines. Negative feedback slows down processes and maintains homeostasis, for example when the production of thyroid stimulating hormone stimulates the thyroid gland to produce thyroid hormones such as thyroxine. As thyroxine levels rise, this inhibits further production of thyroid stimulating hormone, slowing production and maintaining an optimal level in the blood.
Hormones can be excreted via the kidneys in the urine. Some hormones have a short life (oxytocin has a half-life of a few minutes), while others are longer lasting, such as steroid hormones like the oestrogens and cortisol.
The hypothalamus is a small but complex region at the base of the brain that acts as the ‘control centre’, linking the nervous and endocrine systems. It receives information from the brain and organs, monitoring levels of chemicals in the body and responding, controlling hormone release from the endocrine glands. It produces both releasing and inhibiting hormones that act on the pituitary gland (Table 3), affecting pituitary hormone production.
Hypothalamus hormone | Anterior pituitary gland hormone released/inhibited | Target organ or tissues | Action |
---|---|---|---|
Growth-hormone-releasing hormone | Growth hormone | Many (including bones, muscles, organs) | Stimulates growth of body cells, facilitates energy to the fetus |
Growth-hormone-release-inhibiting hormone | Inhibits release of growth hormone, thyroid-stimulating hormone, prolactin | Many | Inhibits hormone production in many parts of the body |
Thyrotropin-releasing hormone | Thyroid-stimulating hormone and prolactin | Thyroid gland | Stimulates thyroid hormone release |
Corticotropin-releasing hormone | Adrenocorticotropic hormone | Adrenal cortex | Stimulates corticosteroid release |
Prolactin-releasing hormone (theoretical) | Prolactin | Milk-producing cells in the breasts | Stimulates milk production |
Prolactin-inhibiting hormone (dopamine) | Inhibits release of prolactin | Milk-producing cells in the breasts | Inhibits milk production |
Gonadotrophin-releasing hormone | Follicle-stimulating hormone Luteinising hormone | Gonads: ovaries | Stimulates ovulation |
The pituitary gland, situated at the base of brain below the hypothalamus, plays a vital role in homeostasis. It has anterior and posterior lobes, a rich blood supply and is physically connected to the hypothalamus by nerve fibres in the pituitary stalk. The anterior pituitary, sometimes called the ‘master endocrine gland’, secretes six key hormones in response to hormones released from the hypothalamus (Table 3). In pregnancy, the anterior pituitary increases in size by approximately 30–50%, mainly because of an increase in lactotrophs (prolactin-releasing cells) (Glezer and Bronstein, 2022). It is susceptible to blood loss, particularly in pregnancy, as it is vulnerable to vasospasm following a sudden decrease in arterial blood supply, for example following haemorrhage, which can lead to the endocrine disorder Sheehan's Syndrome (hypopituitarism). The posterior lobe secretes two hormones, oxytocin and anti-diuretic hormone, which are both produced in the hypothalamus but stored in and released from the posterior lobe; it does not manufacture hormones itself.
Anterior pituitary hormones
Anterior pituitary hormones are numerous and include follicle stimulating hormone and luteinising hormone. Follicle stimulating hormone stimulates the ovary to produce a Graafian follicle containing a mature ovum in females and stimulates sperm production in males. Luteinising hormone triggers ovulation and maintains the corpus luteum, which develops from an ovarian follicle. This secretes progesterone and relaxin and is essential for establishing and maintaining a pregnancy.
Production of prolactin is stimulated by several hormones produced in the hypothalamus, such as anti-diuretic hormone and gonadotrophin-releasing hormone; no specific prolactin-releasing hormone has been identified. Prolactin is inhibited by the stress hormone, dopamine, also produced by the hypothalamus. Prolactin receptors are found in the breasts and ovaries but also many other tissues and organs in the body. In non-pregnant females, prolactin leads to breast tenderness and swelling before menstruation.
Levels of prolactin rise from early pregnancy, stimulating the development of structures in the breast essential for lactation. However, prolactin's influence on milk production is inhibited by high levels of oestrogen and progesterone antenatally. These drop abruptly after birth of the placenta, and increased levels of prolactin directs lactocytes in the breast to produce milk, initiating breastmilk production. The prolactin receptor theory suggests that early breastfeeding, soon after birth, increases the number of prolactin receptors in breast tissue, which optimises milk production (Wambach and Watson, 2016). Levels of prolactin are highest at night. If a woman does not breastfeed, prolactin levels drop rapidly and reach non-pregnant levels by around 7 days postpartum.
Growth hormone stimulates cells to grow and divide, determining the growth of bone, tissues, muscles and organs. It stimulates protein synthesis and the use of fats for fuel. In pregnancy, this helps to facilitate the energy supply to the fetus. Growth hormone stimulates the production of insulin-like growth factors in the liver and regulates other metabolic hormones, including thyroid hormones. The secretion of growth hormone follows a diurnal cycle, being highest during sleep, demonstrating the importance of sleep for recovery from illness and injury.
Thyroid-stimulating hormone is produced by the anterior pituitary gland but is also synthesised by the placenta during pregnancy. Its release affects the production of hormones by the thyroid gland.
Adrenocorticotrophic hormone is also produced by the anterior pituitary gland, in response to the release of corticotropin-releasing hormone from the hypothalamus and synthesised by the placenta during pregnancy. Adrenocorticotrophic hormone stimulates blood flow to the adrenal glands and the production of glucocorticoids, such as cortisol, in response to stress, as part of the hypothalamic–pituitary–adrenal axis (Figure 1).
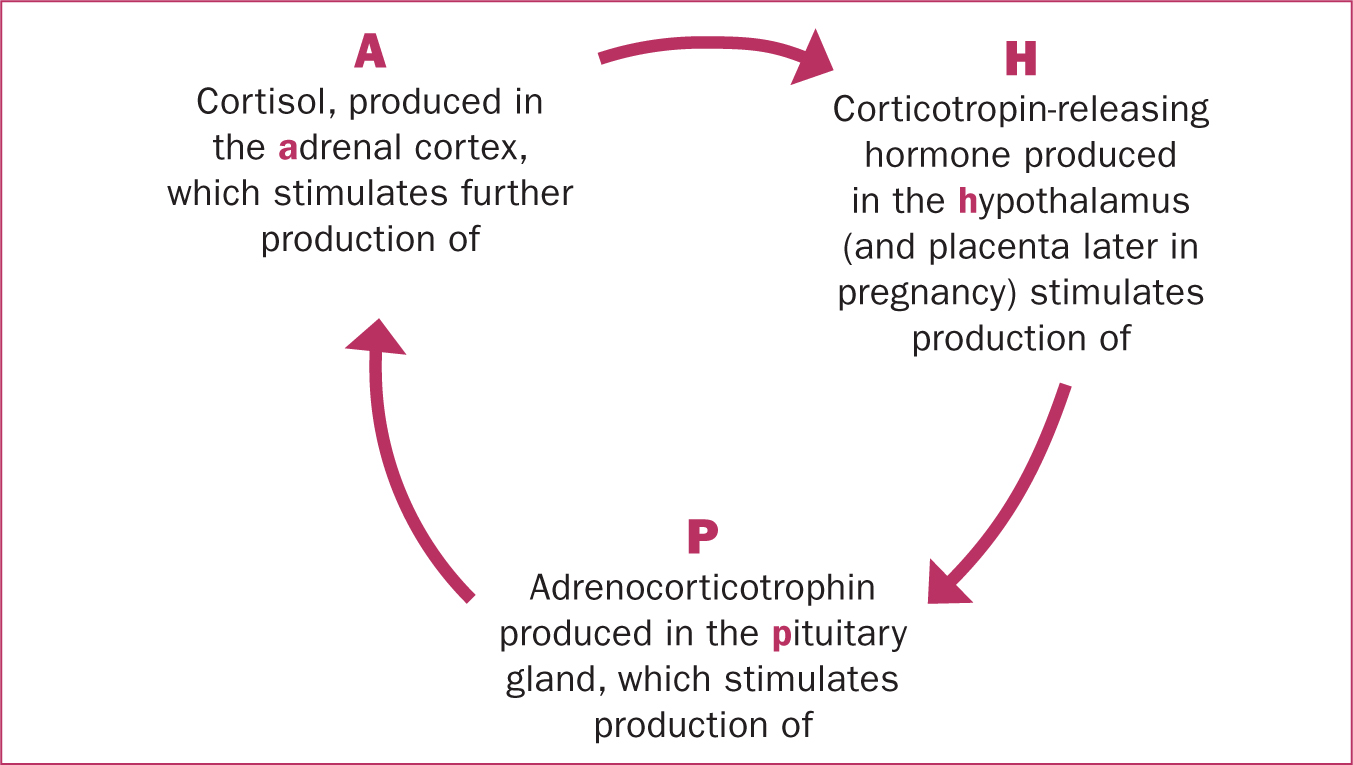
Melanocyte-stimulating hormone affects skin pigmentation. A temporary increase in this hormone and melanin production during pregnancy, stimulated by oestrogen and progesterone, leads to hyperpigmentation in many women, especially those with darker skin. This condition is called melasma, and manifests as dark patches on the skin, usually the face. Pregnant women are also more sensitive to sunburn. Hyperpigmentation also results in the linea nigra, a thin dark line on the skin that runs from the fundus or naval to the pubic area. Hyperpigmentation fades gradually after birth.
Posterior pituitary hormones
Posterior pituitary hormones include oxytocin and anti-diuretic hormone. Oxytocin is a small neuropeptide, or signalling molecule, produced in the hypothalamus but released from the posterior pituitary gland. It is also synthesised in the ovaries, adrenal and thymus glands, and the pancreas. It is produced during sex and childbirth, as well as during social interactions and in response to stress.
Oxytocin reduces stress by activating the parasympathetic nervous system, which promotes calm, healing and growth. It also reduces activity in the sympathetic nervous system, which reduces fear and stress, the production of catecholamines (stress hormones), and increases sociability (Buckley, 2015).
Levels increase gradually during pregnancy, and during the first and second stages of labour, with increasing size and frequency of pulses, with a surge around the time of birth itself, stimulating the ‘fetal ejection reflex’ (Uvnäs-Moberg et al, 2019). Oxytocin levels are particularly elevated in multiparous women during pregnancy and involved in uterine muscle contraction in labour and postnatally, helping to minimise blood loss.
Oxytocin is released in labour due to the stretching of the cervix by the presenting part of the fetus, a neurohormonal feedback mechanism known as Ferguson's reflex, resulting in increased uterine activity. This can be effectively blocked by medical interventions such as epidural analgesia, affecting the progress of labour (Uvnäs-Moberg et al, 2019). Oxytocin release can also be inhibited by stress hormones such as adrenaline, which underpins the use of alternative therapies and coping strategies to promote calm and relaxation during labour and birth. Oxytocin also acts on the myoepithelial cells in the alveoli of the breasts, in response to the infant suckling. This results in their contraction, which releases milk from the lactocytes they surround, into branching ducts which flow to the nipple.
Anti-diuretic hormone, sometimes called vasopressin, acts on the nephrons in the kidneys to reabsorb water and reduce urine production, in response to a decrease in blood fluid volume or blood pressure, monitored by receptors in the hypothalamus. These receptors are reset in pregnancy to accommodate the increased circulating blood volume. Anti-diuretic hormone also acts as a vasoconstrictor to help maintain blood pressure, for example during haemorrhage. Anti-diuretic hormone release is stimulated by pain and drugs such as nicotine and morphine, whereas alcohol and excess water consumption inhibit anti-diuretic hormone release, resulting in diuresis: increased or excessive production of urine.
The thyroid and parathyroid
The thyroid and parathyroid glands are situated in the neck, in front of the trachea and behind the larynx. The parathyroid gland is found on the posterior surface of the thyroid gland. The thyroid is the largest endocrine gland and is extremely vascular. It is supplied with blood through thyroid arteries, branching from the carotid and subclavian arteries.
The glands secrete thyroxine, triiodothyronine and calcitonin from follicular and parafollicular cells. Iodine in the diet is required to produce thyroid hormones. Thyroxine and triiodothyronine are produced in response to thyroid-stimulating hormone being released from the anterior pituitary gland. They are essential for metabolic processes, physical and mental growth, regulating metabolic rate, the metabolism of carbohydrates (via the pancreas), fats and proteins, and also the functioning of the cardiac, nervous and reproductive systems, so have an impact on most cells of the body. The release of thyroxine and triiodothyronine is highest at night, with a negative feedback system controlling levels (high levels of thyroid-stimulating hormone resulting in low secretion of thyroxine and triiodothyronine, and vice versa).
Overall, thyroid function remains normal during most pregnancies, balanced by changes in the metabolism of iodine, requirements for which double in pregnancy. During early pregnancy, the maternal thyroid gland provides the fetus with thyroxine, stimulated by an increase in several hormones, including human chorionic gonadotrophin and oestrogen. As a result, the size and activity of the thyroid glands increase. Increased thyroxine and human chorionic gonadotrophin are linked to nausea and vomiting during this period (Dekkers et al, 2020). Increased thyroxine and triiodothyronine production leads to an increase in metabolic rate, which optimises glucose provision to the fetus. After birth, maternal thyroid hormones take approximately 6–12 weeks to return to their pre-pregnancy levels.
Calcitonin is secreted in response to high levels of calcium and phosphate in the blood. It regulates the levels of these by reducing the amount of calcium released from bone, promoting uptake and increasing the amount secreted by the renal system. Calcitonin levels increase in pregnancy and during breastfeeding, facilitating the transfer of maternal calcium to the fetus/infant while protecting the maternal skeleton from bone loss (Canul-Medina and Fernandez-Mejia, 2019).
Parathyroid hormone is secreted by the parathyroid gland and maintains calcium homeostasis. It works as an antagonist to calcitonin to regulate calcium and phosphate, via the bones and kidneys. It also promotes formation of calcitriol (the active form of vitamin D) in the kidneys, which is involved in absorption of calcium and other minerals in the intestine and may affect the function of the placenta (Wagner and Hollis, 2022). Levels of calcitriol increase in pregnancy.
Adrenal glands
The adrenal gland is situated above each kidney. The gland consists of an inner medulla (part of the sympathetic nervous system) and an outer cortex. The cortex is responsible for the secretion of a range of steroid (cholesterol-based, fat-soluble) hormones: corticosteroids (glucocorticoids and mineralocorticoids) and gonadocorticoids (sex hormones).
Glucocorticoids include cortisol and corticosterone, which have a wide range of effects throughout the body. They maintain homeostasis and regulate metabolism, particularly the metabolism of carbohydrates, to maintain a steady level of plasma glucose. Cortisol can also increase vasoconstriction to maintain blood pressure and fluid balance, increase circulatory efficiency and control sodium and water absorption in the kidneys. Cortisol acts as an anti-inflammatory and can suppress or regulate the immune system. The increase in steroid levels during pregnancy may also lead to an improvement in certain conditions, such as rheumatoid arthritis and eczema.
Glucocorticoids are also produced as part of the body's response to stress. Stress in this context is any challenge that has the potential to disrupt homeostasis. This systemic stress response combines both the suppressive and stimulating actions of glucocorticoids, to provide energy for a ‘flight or fight’ response. The production of glucocorticoids is stimulated by the release of adrenocorticotropic hormone from the anterior pituitary, in response to the release of corticotropin-releasing hormone from the hypothalamus, and following a circadian pattern, increasing after sleep. This is the hypothalamic–pituitary–adrenal axis (Figure 1).
During pregnancy the hypothalamic–pituitary–adrenal axis is stimulated, and levels of glucocorticoids rise in response to oestrogen and progesterone, resulting in increased metabolic activity and cardiac output. This enables body systems to adapt to the requirements of pregnancy. In the second half of pregnancy, the fetal adrenal glands begin to produce cortisol, which promotes maturation of fetal organs, such as the lungs, in preparation for extrauterine life. The fetus, decidua and placenta also stimulate the maternal hypothalamic–pituitary–adrenal axis in late pregnancy; increased cortisol contributes to the onset of labour, increasing the production of prostaglandins and oestrogens, which enhance uterine contractility.
Mineralocorticoids include aldosterone, which is involved in the reabsorption of sodium in kidneys, sweat and saliva, and the removal of hydrogen ions in urine, to maintain water/electrolyte balance. Aldosterone is part of the renin-angiotensin mechanism, which controls blood volume and pressure and the amount of water and electrolytes in extracellular fluid by affecting sodium and potassium concentrations. In pregnancy, levels are increased because of oestrogen and progesterone.
Gonadocorticoids are a group of structurally related sex hormones, including oestrogens, androgens and progesterone. All are produced by both men and women, but in different amounts. They are involved in changes at puberty, being produced by the gonads: the testes in the male and the ovaries in the female. Androgens, such as testosterone, facilitate the maintenance of male characteristics, fertility and libido in both men and women, oestrogens such as oestradiol facilitate the maintenance of female characteristics and fertility.
In pregnancy, levels of oestrogens and progesterone rise significantly, being produced initially by the corpus luteum, then the placenta and fetus. This rise ensures the continuation of pregnancy by facilitating uterine growth, enhanced blood supply and preparation of the breasts for lactation. Oestrogen stimulates the formation of oxytocin receptors on uterine muscle (myometrium), to prepare the uterus for labour and birth, and acts on the thyroid gland to increase production. Progesterone increases threefold by end of pregnancy. It has many functions, including relaxing smooth muscle, such as blood vessels, and in the gastrointestinal and renal systems. Increased progesterone delays gastric emptying, which may be linked to nausea and vomiting in early pregnancy. It acts as an immunosuppressant and stimulates the formation of the decidua (the maternal part of the placenta) from the endometrium and inhibits uterine action until the end of pregnancy.
The adrenal medulla is part of the sympathetic nervous system. It produces the catecholamines (stress hormones) adrenaline and noradrenaline, along with dopamine (which can be modified to form adrenaline). Dopamine is also produced by the hypothalamus and suppresses the secretion of prolactin from the pituitary gland. This is part of the body's acute stress response, a physiological state of hyperarousal in response to short-term stress that threatens homeostasis. This triggers a response in the hypothalamus, resulting in the release of hormones from the pituitary and adrenal glands, including adrenocorticotropic hormone, adrenaline and cortisol. These increase muscular contraction, cardiovascular and respiratory function and decrease digestion.
Catecholamines stimulate the liver to produce glucose and prepare the body for action: ‘flight or fight’. Neuroendocrine evidence from animal and human studies (Carter and Kingsbury, 2022) suggests that oxytocin, in conjunction with female reproductive hormones such as oestrogen and endogenous opioids, may be responsible for downregulating this stress response, activating the parasympathetic nervous system and reducing activity in the sympathetic nervous system. However, oxytocin release itself can be inhibited by catecholamines, impacting the progress of labour and birth.
Other hormones
Situated in between lungs, the thymus gland secretes hormones such as thymopoietin, thymosin and thymulin to stimulate production of T cells (T lymphocytes) in the bone marrow, as part of an immune response. Increased levels of some T cells help to balance the body's immune response to pregnancy, protecting the fetus (Hellberg et al, 2019).
Melatonin is produced in the pineal gland in the brain, but also in a wide range of organs and tissues in the body, including the uterus, placenta and immune system cells. It is secreted in a circadian manner, with highest levels at night, and its secretion is stimulated by darkness and inhibited by light. It has an impact on biorhythms, including daily variations in temperature, sleep and appetite, and has a critical role in homeostasis. Melatonin has antioxidant and anti-inflammatory properties and impaired levels have been linked to diseases that develop in adult life, through the concept of fetal programming (Kvetnoy et al, 2022).
Endocrine cells exist in the many organs and tissues in the body, including the ovaries, testes, placenta, pancreas and digestive system. Individual cells can produce hormones too but the function of these is not always clear. The pancreas is made up of both exocrine and endocrine cells. In the Islets of Langerhans, different cells have different functions. This includes alpha cells, which secrete glucagon when blood glucose levels are low. Glucagon converts glycogen, stored in the liver and muscles, into glucose. Beta cells secrete insulin when blood glucose levels are high. Insulin converts glucose into glycogen for storage, increases the uptake of glucose by cells, especially muscle cells, and the storage of fats in adipose tissue. Other cells in the pancreas secrete hormones that regulate the secretion of glucagon, insulin and digestive enzymes.
Hormones in pregnancy
Relaxin is released initially by the corpus luteum and later produced by the placenta and decidua. Levels are highest at the end of the first trimester. Relaxin stimulates endometrial decidualisation (changes to the cells of the endometrium in preparation for, and during, pregnancy) and other changes to facilitate successful implantation of the embryo and development of the placenta. It facilitates many of the cardiovascular and renal adaptations of pregnancy and inhibits uterine contractions, having vasodilatory and anti-fibrotic properties (Sarwar et al, 2017). Relaxin relaxes pelvic ligaments and is thought to soften the symphysis pubis, to allow the growth of the uterus into the abdomen.
Human chorionic gonadotrophin is initially produced by embryonic trophoblast cells and then the placenta. It stimulates the thyroid gland, and affects appetite, thirst and fluid balance through anti-diuretic hormone release. Human chorionic gonadotrophin also stimulates ovulation and progesterone production in the corpus luteum in early pregnancy until the placenta takes over. Increased levels of human chorionic gonadotrophin are an early positive sign of pregnancy: the presence of human chorionic gonadotrophin can be detected in urine two weeks after conception, which is used by some pregnancy tests. Low levels are associated with early pregnancy failure.
Human placental lactogen is also produced by trophoblast cells and the placenta and is only present during pregnancy. Like growth hormone, human placental lactogen modifies the metabolic state of the mother to facilitate the energy supply to the fetus. It is antagonistic to insulin, and has a diabetogenic effect, increasing maternal metabolism and the use of fat for energy, and reducing the uptake of glucose by cells, so blood glucose levels remain higher for fetal use. Human placental lactogen increases as human chorionic gonadotrophin falls, in parallel with the size of the placenta. Low levels are associated with pregnancy failure. Human placental lactogen mimics prolactin and competes with it for receptors on the breast, but a fall in levels after birth of the placenta is associated with a rise in prolactin.
Prostaglandins are an example of ‘eicosanoids’ or local hormones, present and synthesised in most tissues, including the myometrium of the uterus, the cervix, ovaries, placenta and fetal membranes. They act on the tissue that secretes them (autocrine) or adjacent tissues (paracrine). Their functions are wide ranging; prostaglandins are short-lived vasodilators, inhibit blood clotting, and influence muscle contraction and the body's inflammatory response. They also stimulate uterine contractions, with synthetic prostaglandins having been used to induce labour since the 1960s.
Serotonin is produced in the brainstem and the intestine and has a wide range of functions, including regulation of mood, appetite, digestion and sleep. Serotonin also has some cognitive functions, influencing memory and learning. Drugs that alter serotonin levels are used in treating depression and anxiety. Levels rise in pregnancy: the placenta provides serotonin to the fetal brain, which may be important for normal brain development (Rosenfeld, 2021).
Disorders of the endocrine system
Diabetes
In the Islets of Langerhans of the pancreas, alpha cells secrete glucagon when blood glucose levels are low, which converts glycogen, stored in the liver and muscles, into glucose. Beta cells secrete insulin when blood glucose levels are high. Insulin converts glucose into glycogen for storage, and also increases uptake of glucose by cells, especially muscle cells, and the storage of fats in adipose tissue.
Diabetes mellitus can be defined as a group of metabolic diseases characterised by hyperglycaemia (high blood glucose) resulting from defects in insulin secretion, insulin action or both (Mukhtar et al, 2020). Diabetes is one of the most common endocrine disorders, affecting women of reproductive age. The three major types of diabetes are shown in Table 4.
Type | Onset/diagnosis | Cause/risk factors | Key characteristics | Treatment/management |
---|---|---|---|---|
Type 1 | Childhood | Viral illness in childhood | Autoimmune, destruction of cells in pancreas: insulin production reduced/ceases | Insulin dependent |
Type 2 | Adulthood | Obesity, age, ethnicity, family history | Cardiometabolic; insulin resistance, insufficient insulin produced | Diet, exercise, oral treatment, insulin if required |
Gestational | During pregnancy | More common in those at risk of type 2; obesity, ethnicity, family history | Insufficient additional insulin produced to compensate for increased resistance in pregnancy |
Diet, exercise, oral treatment, insulin if required |
Type 1 diabetes mellitus accounts for about 8% of all diabetes in the UK (Diabetes UK, 2019). Its cause is unclear; however, a childhood virus (Coxsackie B4) has been implicated in the onset of type 1 diabetes mellitus, with a peak incidence around 12 years (Nekoua et al, 2022). The virus destroys cells in the pancreas and triggers an autoimmune response in those genetically susceptible. Progressive destruction of beta cells occurs, often over an extended period, resulting in an almost complete lack of insulin production, as well as impaired alpha cell function, leading to an excess of glucagon. This results in hyperglycaemia, causing damage at cellular and organ levels. Insulin treatment is essential to prevent cardiovascular and microvascular damage resulting from hyperglycaemia.
Type 2 diabetes mellitus accounts for about 90% of diabetes in the UK (Diabetes UK, 2019). Onset is usually later in life, associated with obesity, age and ethnicity. Rates of type 2 diabetes mellitus in pregnancy are increasing (National Institute for Health and Care Excellence, 2020). In type 2, there is increased insulin resistance and insulin has reduced capability to influence cellular uptake of glucose, because of a reduction in insulin receptors on cells. Cell destruction in the Islets of Langerhans also leads to reduced insulin production. These result in sustained hyperglycaemia. Type 2 diabetes mellitus is associated with an increased risk of long-term health complications.
Gestational diabetes mellitus is defined as any degree of glucose intolerance with onset or first recognition during pregnancy (McIntyre et al, 2019). It has been estimated that globally, in 2021, 13.9% of live births were affected by gestational diabetes mellitus (International Diabetes Federation, 2021). The prevalence of women with gestational diabetes mellitus is increasing, because of higher rates of obesity and older women becoming pregnant. After birth, gestational diabetes mellitus usually disappears; however, the recurrence rate in a subsequent pregnancy is around 50% (Schwartz et al, 2015). In addition, women with gestational diabetes mellitus have a substantially increased risk of type 2 diabetes mellitus (up to 17-fold compared to women without gestational diabetes mellitus), with the risk highest during the 3–6 years after gestational diabetes mellitus and in women aged less than 40 years but remaining markedly elevated thereafter (Song et al, 2018). Risk factors for gestational diabetes mellitus are shown in Box 1.
Risk factors for gestational diabetes
Note: Glycosuria during pregnancy may indicate gestational diabetes mellitus and warrants further testing. Smoking and significant inter-pregnancy weight gain also increase risk
Pregnancy has a diabetogenic effect on the body, altering glucose metabolism. This impacts maternal metabolism, especially in the third trimester, with progressive insulin resistance as a result of the placental hormones oestrogen and human placental lactogen, which decrease insulin efficiency. The resulting hyperglycaemia increases plasma glucose availability to the fetus, favouring fetal growth. This increase in insulin resistance is normally countered by an increased production of insulin, stimulated by progesterone. Diabetes with onset during pregnancy results from a reduced capacity to compensate for this insulin resistance, perhaps as a result of a genetic predisposition for diabetes. Glucose metabolism becomes unstable and pre-existing diabetes becomes more difficult to control too. Risks associated with diabetes during childbearing for both the woman and fetus/neonate are summarised in Table 5.
Increased risks for the women | Increased risks for the fetus/neonate |
---|---|
Perinatal health | |
Pregnancy-induced hypertension Pre-eclampsia | Fetal growth restriction, with pre-eclampsia or nephropathy |
Worsening of retinopathy and nephropathy (types 1 and 2 diabetes mellitus) | Fetal and congenital malformations |
Polyhydramnios (excess amniotic fluid) | Fetal macrosomia (excessive growth) |
Premature labour and birth | Neonatal asphyxia/respiratory distress |
Induction of labour | Birth injury because of shoulder dystocia, eg Erb's Palsy |
Instrumental/operative birth | Neonatal hypoglycaemia |
Birth injury (eg third degree perineal tear) | Admission to neonatal unit |
Infection | Polycythaemia leading to neonatal jaundice |
Not breastfeeding successfully | Miscarriage, stillbirth and neonatal death |
General health | |
Type 2 diabetes mellitus after gestational diabetes mellitus | Type 2 diabetes mellitus as an adult |
Hypertension, cardiovascular disease | Metabolic and cardio-vascular disease in adulthood |
Non-alcoholic fatty liver disease | Obesity |
Neuropathy/retinopathy/nephropathy | Neuropsychiatric disease, neurodevelopmental impairment |
Thyroid disorders
Hypothyroidism consists of a lack of triiodothyronine and thyroxine and occurs either because of a defect in the thyroid gland or in the control pathway for thyrotropin-releasing hormone or thyroid-stimulating hormone. Hypothyroidism reduces fertility if untreated and is associated with increased thyrotropin-releasing hormone and thyroid-stimulating hormone, which raise levels of prolactin in the blood (hyperprolactinaemia), preventing ovulation. It is also a relatively common pregnancy-related thyroid disorder, affecting approximately 1–2% of all pregnant women (Li and Chan, 2020).
A common cause of hypothyroidism in the UK is chronic autoimmune thyroiditis, with or without goitre (Hashimoto's thyroiditis). Hypothyroidism can also result from sub-acute thyroiditis, a temporary inflammation of the thyroid gland triggered by viral infections such as mumps or influenza, or a result of thyroidectomy or radioactive iodine treatment. Hypothyroidism during pregnancy can also occur because of treatment for Grave's disease. Autoimmune thyroiditis is familial and is associated with other autoimmune disorders such as type 1 diabetes mellitus.
Symptoms of hypothyroidism include weight gain, constipation, alopecia, dry skin, lethargy and cognitive impairment, but many women are asymptomatic when diagnosed. Hypothyroidism is confirmed through the thyroid function test, with high thyroid-stimulating hormone and normal or low fetal thyroxine and fetal triiodothyronine levels. Pregnancy complications include pregnancy-induced hypertension and gestational diabetes mellitus, and impaired brain development and physical growth in the fetus, resulting in low birth weight. There may also be an association between thyroid dysfunction and depression during pregnancy and in the postpartum period (Schmidt et al, 2022).
Hyperthyroidism (thyrotoxicosis) results from excessive production of thyroid hormones. Severe hyperthyroidism is associated with infertility, but pregnancy may occur if the condition is mild or successfully treated. Hyperthyroidism in pregnancy is mainly caused by transient gestational hyperthyroidism or Graves' disease (autoimmune hyperthyroidism); other causes are rare (Nguyen et al, 2018). Transient gestational hyperthyroidism is usually mild and affects 2–15% of pregnancies, occurring in the first half of pregnancy (Karras and Tzotzas, 2022). It is caused by stimulation of thyroid follicular cells by placental human chorionic gonadotrophin, structurally like thyroid-stimulating hormone, which peaks around 10 weeks' gestation. As women with hyperemesis gravidarum tend to have high levels of human chorionic gonadotrophin, they are at increased risk. It resolves by 20 weeks' gestation and treatment is not usually required.
Graves' disease affects about 1 in 500 pregnant women (Panaitescu 2021). Thyroid-stimulating antibodies activate thyroid-stimulating hormone receptors, leading to increased production of thyroid hormones. In some cases, Graves' disease is associated with other autoimmune conditions such as type 1 diabetes mellitus and pernicious anaemia as a result of vitamin B12 deficiency. Trophoblastic tumours such as hydatidiform mole may rarely cause hyperthyroidism, as well as the overtreatment of hypothyroidism. Symptoms include heat intolerance, sweating, palpitations, weight loss, hypertension, insomnia and anxiety. The condition is confirmed through the thyroid function test, with raised fetal thyroxine and low thyroid-stimulating hormone levels.
In women with pre-existing disease, the condition may get worse in the first trimester but generally improves throughout the rest of the pregnancy. Graves' disease tends to improve in pregnancy; 30–40% of women may be able to stop antithyroid medication after 30 weeks' gestation (Nguyen et al, 2018). Hyperthyroidism is associated with severe adverse effects for mother and fetus, such as pre-eclampsia and heart failure in the woman, and growth restriction, miscarriage, premature birth and stillbirth/neonatal death. There are also risks of the transfer of thyroid antibodies, resulting in neonatal thyroid dysfunction. This may get worse initially, and may require temporary treatment, but resolves within 3 months of birth. Treatment of hyperthyroidism may also result in fetal hypothyroidism.
Conclusions
Midwives require a working knowledge of normal physiology and the most common pathophysiology and diseases to provide individualised care to woman. They need to be aware of how the risk of serious morbidity and mortality during pregnancy from disorders of the endocrine system can be reduced. Pregnancy may exacerbate pre-existing conditions and some disorders may have longer term or lifelong consequences. There are many diseases affecting the endocrine system and pregnancy is an added risk factor if a woman has any pre-existing endocrine condition, or one develops during pregnancy or the postnatal period. Treatment and medication for endocrine conditions should always follow clinical protocols, considering any contraindications in pregnancy. A holistic and multidisciplinary approach, considering the woman's wishes, providing psychosocial support and clearly outlining the risks and care plan, will result in the best possible outcomes for women and babies.