In parthernship with the editors for Fundamentals of Maternal Anatomy and Physiology (Peate and Leader, 2024), and Fundamentals of Maternal Pathophysiology (Leader and Peate, 2024), this series provides an evidence-based summary of the cardiac, respiratory and endocrine systems. This second article explores the maternal respiratory system, outlining the anatomy and key physiological adaptations in pregnancy, and summarising the key pathophysiological conditions that may occur.
The respiratory system ensures the oxygen needed for cellular respiration is taken from the air we breathe in to all the cells of the body, where cellular respiration takes place. Many disorders can affect the respiratory system, from the common cold to more severe conditions such as asthma, tuberculosis, pneumonia and pulmonary embolism. It is important for midwives to be aware of the mechanisms of ventilation and respiration and changes in the respiratory function of a woman in their care that require appropriate action.
Overview of the respiratory system
The respiratory system provides the exchange of gases needed for cellular respiration. Air is inhaled from the atmosphere into the lungs, oxygen is then transported via the cardiovascular system to the tissues. Carbon dioxide, metabolic waste from respiration, is returned to the lungs and excreted in the air expired. The demand for oxygen and removal of carbon dioxide is greater during pregnancy, labour and the postnatal period because of increased workload. However, a healthy respiratory system can cope with these demands (Coad et al, 2019).
Structurally, the respiratory system consists of two parts: the upper respiratory system (the nose, pharynx, larynx and associated structures) and the lower respiratory system (the trachea, bronchi and lungs). Functionally, the respiratory system can also be separated into two parts: the conducting part (consisting of a series of interconnecting cavities and tubes, outside and within the lungs, that filter, warm and moisten air, conducting it into the lungs) and the respiratory part (consisting of tissues in the lungs where gas exchange occurs) (Tortora and Derrickson, 2023).
The upper respiratory system
Air enters the airway through the nose or mouth. The nose is made up of bone and cartilage and houses a large, irregular shaped cavity divided in two by the nasal septum, with two anterior openings called the nares or nostrils. Ciliated columnar epithelial cells, containing mucous-secreting goblet cells, line the nasal cavity. Air entering via the nasal cavity is filtered by hairs in the nasal passages, warmed by contact with the tissues in the nasal cavity and moistened by cells in the mucous membrane of the nasal cavity. Two posterior nares open into the pharynx. The ciliated cells in the nasal cavity provide a gentle current moving any trapped mucus towards the pharynx, where it is swallowed, passed to the stomach and digested. Olfactory receptors are in the superior mucosa of the nasal cavity, detecting odours as part of the sense of smell.
The pharynx is a funnel-shaped tube, beginning at the posterior opening of the two nasal passageways and ending at the larynx, made up of three regions: the nasopharynx, oropharynx and laryngopharynx. Water and food pass through the pharynx in addition to air. The pharynx warms and moistens air further, protects the tympanic membrane or eardrum from changes in atmospheric pressure, acts as a resonance chamber for sound and produces antibodies for infection prevention.
The nasopharynx extends from the nasal cavity to the level of the soft palate of the mouth, containing the two openings of the auditory or Eustachian tubes leading to the middle ears. The pharyngeal tonsil or adenoids are also found in the upper region of the nasopharynx. The mucosa of the nasopharynx consists of ciliated columnar epithelium and is continuous with the lining of the nose.
The oropharynx extends from the inferior tip of the soft palate to the superior tip of the epiglottis, where the palatine and lingual tonsils are situated. Two folds are formed at the lateral walls on each side of the oropharynx, blending with the soft palate. A collection of lymphoid tissue, called the palatine tonsil, can be found between each pair of folds. The mucosa in the oropharynx changes to tougher stratified squamous epithelium and is continuous with the lining of the mouth and oesophagus. This provides protection from food and other materials passing through during swallowing.
At the inferior aspect of the oropharynx, the laryngopharynx can be found. Air and food pass through the laryngopharynx until the tube divides in two: anteriorly, the larynx and trachea transport air into the lungs; posteriorly, the oesophagus transports food and liquid into the stomach. The mucosa in the laryngopharynx also consists of tougher stratified squamous epithelium, continuous with the lining of the mouth and oesophagus.
After the pharynx, air passes first through the larynx onto the trachea, a single tube forming the major airway. Several cartilages are associated with the larynx: the thyroid cartilage, made of hyaline cartilage and the most prominent, the cricoid cartilage, arytenoid cartilages and the epiglottis.
The vocal cords, a pair of folds in the mucous membrane, are in the larynx. The vocal cords can vibrate when air passes over them, causing sound waves, allowing speech.
The lower respiratory system
After the larynx, air enters the trachea then passes through one of two bronchi. The bronchi divide repeatedly into smaller bronchioles (terminal and respiratory), leading to alveoli ducts, before ending in air sacs, called alveoli (Figure 1).
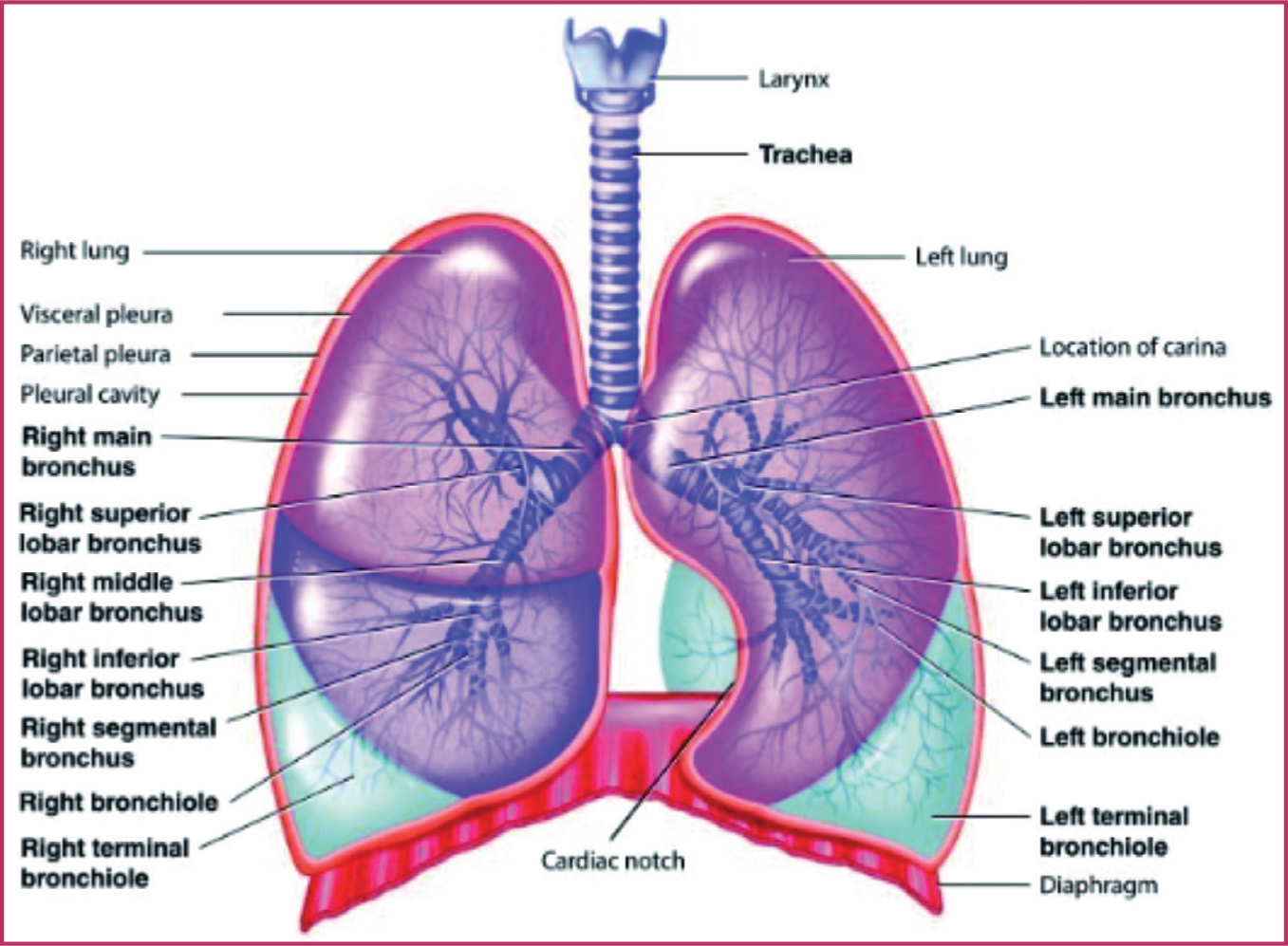
The trachea lies in front of the oesophagus and is a 100–120mm long tube, held open by 16–20 horseshoe- or C-shaped rings of hyaline cartilage. The trachea wall has three layers, an outer fibrous and elastic tissue layer, enclosing the C-shaped rings of cartilage, a middle layer of cartilage, bands of smooth muscle and some areolar tissue, containing blood vessels, lymph vessels and autonomic nerves, and an inner lining consisting of a mucous membrane containing ciliated epithelium (which beat in a wave-like manner, moving mucus, dust and microorganisms upwards and out of the lungs).
The C-shaped cartilage rings are incomplete on the posterior side, next to the oesophagus. These cartilage rings have two main functions: maintaining patency during pressure changes associated with breathing and allowing for slight expansion of the oesophagus during swallowing of food or fluid.
The trachea subdivides into two main branches, the right and left bronchus, dividing repeatedly into smaller and smaller tubes called bronchi and finally, bronchioles. The trachea, bronchi and bronchioles contain smooth muscle, which enables them to constrict. The bronchioles lead ultimately to numerous alveoli, where most gaseous exchange takes place. The right bronchus is wider, shorter and straighter than the left bronchus because of the position of the heart on the left side of the chest.
As the bronchi divide into the smaller bronchioles, the rings of cartilage supporting the airways are replaced by smooth muscle. The rings of cartilage reduce in size as the bronchi divide. The lower bronchioles have no cartilage present, as it would interfere with the exchange of gases and the expansion of lung tissue. For airflow to be regulated in each lung, the amount of smooth muscle in the bronchioles increases as the cartilage decreases. This smooth muscle increases and decreases the diameter of the airways, in response to stimuli, through contraction and relaxation under the control of the autonomic nervous system. The sympathetic nervous system causes bronchodilation and the parasympathetic nervous system causes bronchoconstriction. As the bronchi divide, eventually the smaller passageways are termed terminal bronchioles, then respiratory bronchioles and finally alveoli ducts lead to the alveoli or air sacs.
The lungs are large cone-shaped organs with a broad concave base, found in the thoracic cavity. The heart, in the mediastinum, separates the two lungs. At the mediastinal surface of the lungs, a triangular area can be found, called the hilum. This is where the bronchus, blood vessels (pulmonary artery and veins, and bronchial arteries and veins), nerves and lymphatic vessels enter the lungs. The most superior part of the lungs, the apex, extends just above the clavicles. The base of the lungs is concave and lies just above the diaphragm. The costal surface of the lungs presses against the costal cartilages, intercostal muscles and rib cage.
Each lung is divided into lobes by fissures: the right lung has three distinct lobes (superior, middle and inferior), while the left lung has two distinct lobes (superior and inferior). The lungs are surrounded by a two-layered pleural membrane that secretes pleural fluid from the epithelial cells, aiding the movement of the lungs against the thoracic wall without friction and preventing the pleural layers from separating by surface tension. The membrane covering the surface of the lungs is the visceral or pulmonary pleura and the part of the membrane covering the thoracic wall and diaphragm is the parietal pleura.
The lungs function to oxygenate the blood for the other organs and tissues of the body. However, they need their own blood supply. The pulmonary artery delivers deoxygenated blood to the lungs, where it is oxygenated in the pulmonary network of capillaries surrounding the alveoli. The oxygenated blood returns to the left atrium of the heart via the pulmonary veins. The bronchial arteries branch from the aorta and provide the lungs with oxygenated blood for their own cellular respiration. Deoxygenated blood leaves the lungs via the bronchial veins and is deposited in the superior vena cava before entering the right atrium.
The lungs provide a large surface area by the presence of an estimated 200–400 million alveoli or air sacs. Each have a network of capillaries surrounding them and have a thin exchange surface. There are only two layers of cells, known as the respiratory membrane, between the air in the alveolus and the blood in the capillaries, through which oxygen and carbon dioxide diffuse in a process called gaseous exchange (Figure 2). These layers are the alveolar epithelium (squamous epithelial cells) and the cells forming the capillary wall. Alveoli are minute bubble-like air sacs lined with moisture, and are liable to collapse, causing their sides to stick together because of surface tension. Septal cells in the alveolar epithelium secrete surfactant, which greatly reduces the surface tension of the alveoli and keeps them open during expiration, thereby preventing their collapse. Oxygen and carbon dioxide are exchanged at the respiratory membrane by a process called diffusion, relying on pressure gradients between the lungs and atmospheric pressure.
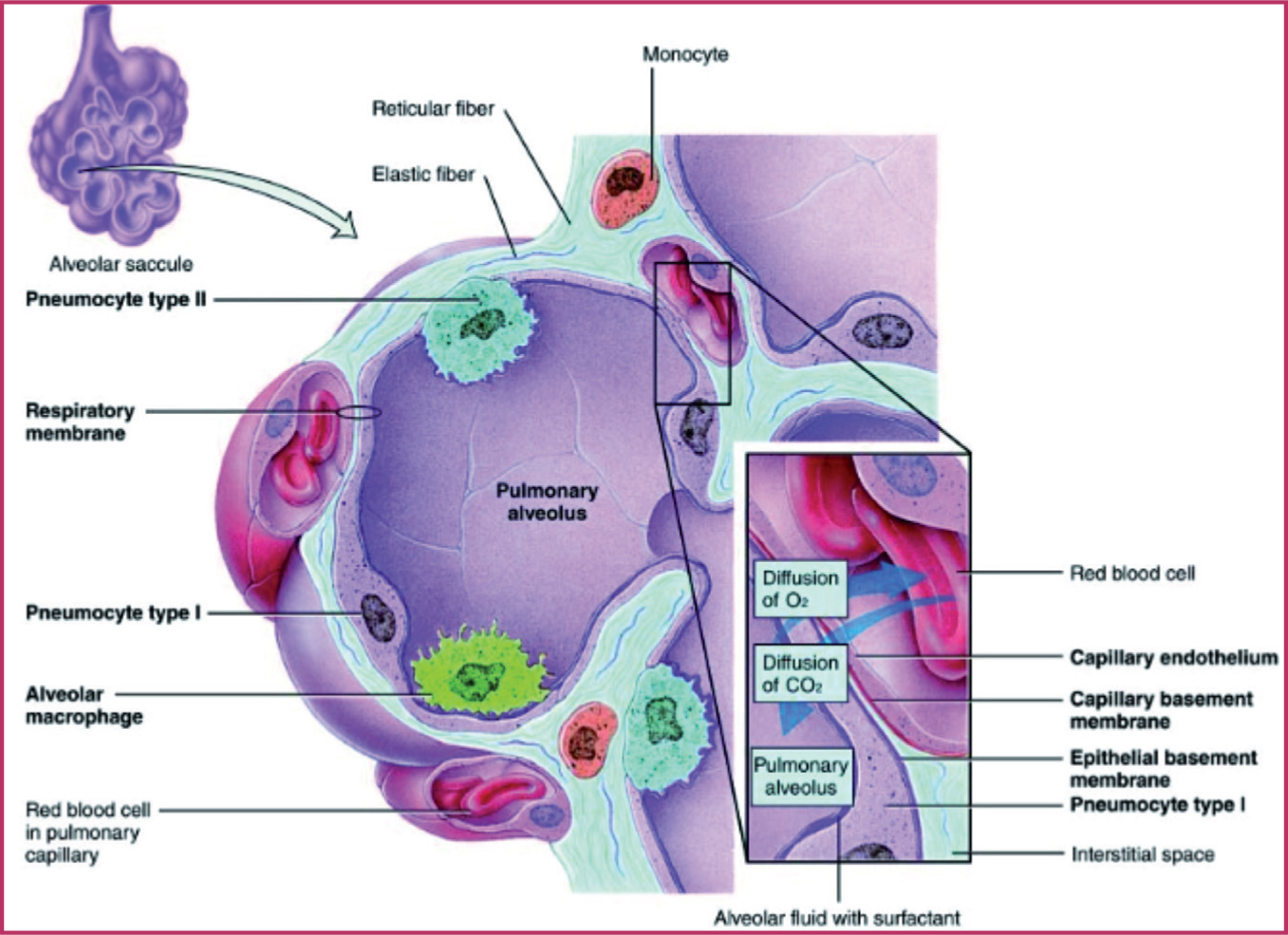
The respiratory muscles support lung function. Forming the floor of the thoracic cavity, separating it from the abdominal cavity, is the strong dome-shaped muscle of the diaphragm. The diaphragm muscle fibres radiate from a central tendon, attaching to the vertebral column, sternum and lower ribs. Contraction of this muscle causes the diaphragm to move downwards and flatten, lifting the rib cage and enlarging the thoracic cavity, causing a decrease in pressure in the thoracic cavity. During contraction, the central tendon moves downwards to be level with the ninth thoracic vertebrae, when the diaphragm muscle is in its relaxed state, the central tendon is level with the eighth thoracic vertebrae.
The phrenic nerves supply the diaphragm. A total of 11 pairs of intercostal muscles lie between the 12 pairs of ribs and are accessory muscles of respiration. There are two layers: the external and internal intercostal muscles. The external intercostal muscles are involved in inspiration, extending downwards and forwards from the lowest edge of the rib above to the uppermost edge of the rib below. The internal intercostal muscles are involved in expiration during phases of activity, extending downwards and backwards from the lowest edge of the ridge above to the uppermost edge of the rib below. They lie at right angles to the external intercostal muscles. The role of intercostal muscles is to primarily stabilise the rib cage during expansion of the thoracic cavity, rather than having any major function in the expansion itself. However, during physical exertion or upper airway obstruction, when there is an increased need for oxygen, the intercostal muscles help to enlarge the rib cage allowing for further lung expansion. As the first rib is fixed, when the external intercostal muscles contract they move the ribcage upwards and outwards towards the first rib.
The process of breathing, or pulmonary ventilation, moves air in and out of the lungs. The average adult has a respiratory rate of 12–15 breaths per minute. Breathing is made up of two phases: inspiration (movement of air into the lungs) and expiration (movement of air out of the lungs). Gases flow from regions of high pressure to regions of low pressure until the pressure gradient is equal, according to Boyle's law (Record and Beecroft, 2022). For inspiration to occur, the gas pressure in the alveoli must be less than that in the atmosphere; for expiration, the gas pressure in the alveoli must be greater than that in the atmosphere (Lumb and Thomas, 2020).
Inspiration occurs by enlarging the thoracic cavity (the intercostal muscles contract, drawing the rib cage upwards and outwards, and the diaphragm contracts and moves downwards), increasing the volume of the lungs. As the ribcage and diaphragm move, the parietal and visceral pleura, and consequently the lungs, are pulled with them, causing lung expansion. This reduces the gas pressure in the alveoli to below atmospheric pressure, creating a pressure gradient, drawing air into the lungs until the alveolar pressure equals that of atmospheric pressure. Energy is needed for muscle contraction; inspiration is an active process. During expiration, the respiratory muscles relax, reducing the volume of the thoracic cavity resulting in an elastic recoil of the lungs and an associated temporary rise in alveolar pressure to above atmospheric pressure occurs. This rise in alveolar pressure causes air to be pushed out of the lungs. Expiration is usually a relatively passive process in resting conditions, as energy is not usually required (Lumb and Thomas, 2020). The lungs contain a small volume of air after expiration and are prevented from collapse by the pleura. Three factors can affect ventilation:
In addition, a range of terms are used to describe lung ventilation, summarised in Box 1.
Glossary of terms associated with lung ventilation
Gaseous exchange
Diffusion is the movement of a substance across a semi-permeable membrane from an area of high concentration to an area of low concentration to reach equilibrium. Diffusion determines the movement of gases in external and internal respiration. Air is a mixture of gases including oxygen, nitrogen, carbon dioxide and water vapour. Inspired and expired air contain different percentages of these gases. The pressure exerted by an individual gas in a mixture is known as its partial pressure, eg PO2 or PCO2. Alveolar air is saturated with water vapour and therefore has a reduction in partial pressures of the other gases compared to atmospheric air. It contains decreased oxygen and increased carbon dioxide levels.
Gaseous exchange (external respiration) between the alveoli and surrounding blood capillaries is constant. External respiration is the exchange of gases at the alveolar surface between the alveoli and blood capillaries that surround them. Blood arriving at the alveoli in the pulmonary artery from tissue cells of the body has high levels of carbon dioxide (5.8kPa) and low levels of oxygen (5.3kPa) compared to alveolar air (Wheeldon, 2020). Carbon dioxide diffuses down a concentration gradient into the alveoli, oxygen diffuses from the alveoli to the blood in the capillaries. Blood leaving the alveoli has equal carbon dioxide and oxygen concentrations to that of the air in the alveolar space.
Internal respiration is the exchange of gases at the level of the cells in the tissues between the systemic capillaries and tissue cells. Blood arriving at the cells in the body from the lungs has high levels of oxygen (13.3kPa) and low levels of carbon dioxide (5.3kPa) (Karbing and Rees, 2020). This creates a concentration gradient between the capillaries and tissue cells of the body. Oxygen diffuses down a concentration gradient from the capillaries into the cell; carbon dioxide diffuses from the cells into the blood in the capillaries.
The two gases involved in respiration, oxygen and carbon dioxide, are carried in the blood in different ways. Oxygen is mostly (99%) transported attached to haemoglobin molecules in the erythrocytes in the form of oxyhaemoglobin. This reaction is reversible and certain conditions, such as reduced oxygen levels, acidic pH and increased temperatures, cause oxygen to dissociate from haemoglobin and therefore be released. In this way, oxygen is released to the cells where it is needed most. A small amount of oxygen is also carried dissolved in the plasma. The equilibrium between oxygen and haemoglobin can be represented by the oxygen-haemoglobin dissociation curve (Figure 3). The S-shaped curve demonstrates the relationship between the partial pressure of oxygen and the percentage of haemoglobin saturated with oxygen. The left-hand side of the curve is steep showing that in low partial pressure of oxygen, haemoglobin releases oxygen to the cells of the body. Small changes in partial pressure of oxygen at these low levels result in larger changes in haemoglobin saturation. The right-hand side of the curve flattens off, showing that in high partial pressures of oxygen, there are smaller changes in haemoglobin saturation. This demonstrates oxygen uptake in the lungs.
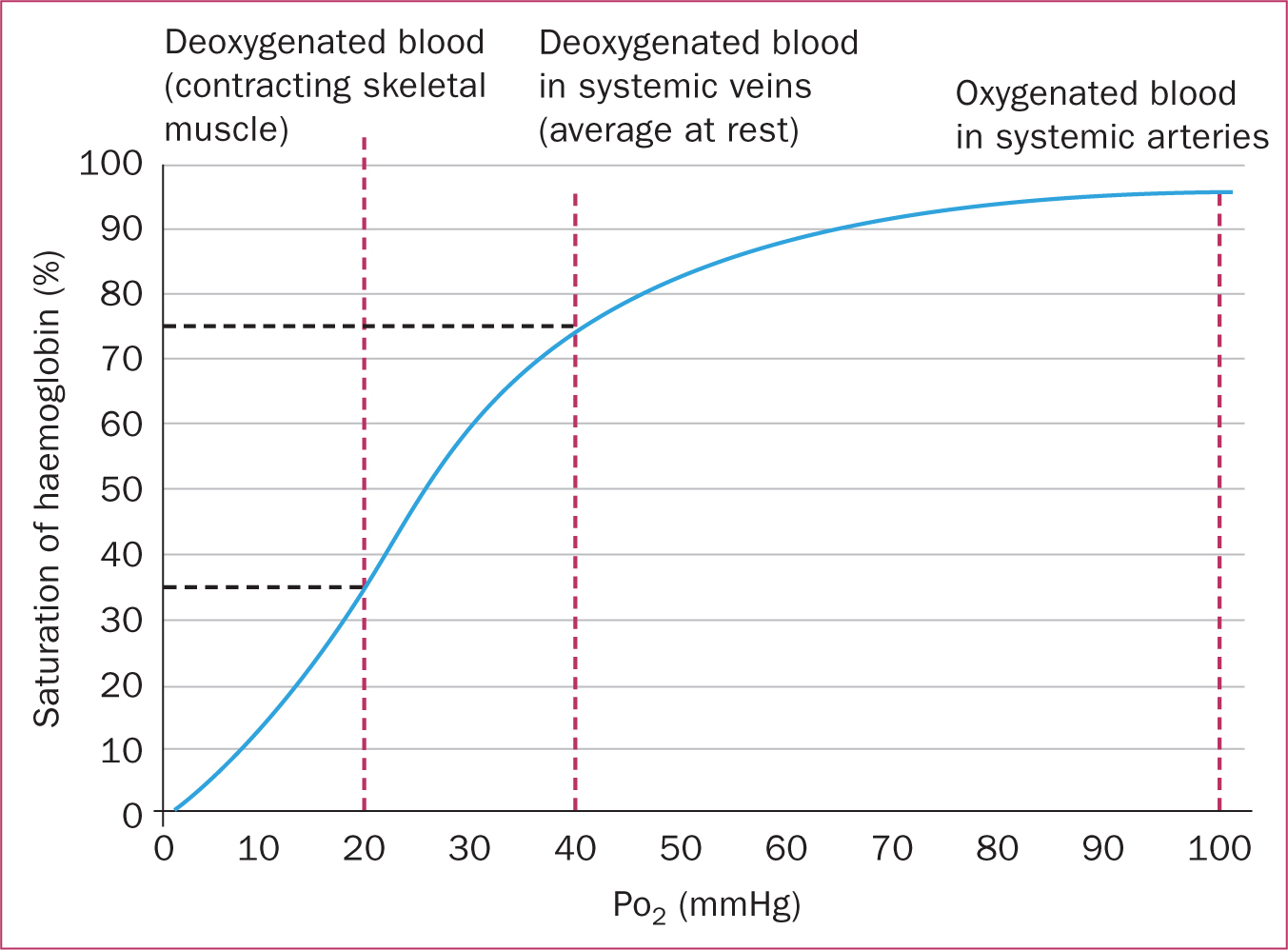
Carbon dioxide is a waste product of metabolism and is mostly (70%) transported in the plasma of blood as bicarbonate ions (HCO3-). Approximately 23% of carbon dioxide is transported in erythrocytes as carbaminohaemoglobin (Humphry and Armstrong, 2022). It does not affect the oxygen-carrying capacity of erythrocytes, as carbon dioxide is bound to the globin rather than the haem part of haemoglobin (Rankin, 2017). Approximately 7% of carbon dioxide is carried in simple solution in plasma (Humphry and Armstrong, 2022).
Control of respiration
Respiration is normally under involuntary control, as the body responds to varying changes in conditions. Voluntary control can be exerted as the need arises, for example, when speaking. However, involuntary control resumes if carbon dioxide levels in the blood rise (hypercapnia), disrupting homeostasis. The respiratory centre is a group of nerves found in the medulla oblongata in the brainstem, which controls the rate and depth of breathing via inspiratory neurones. The pneumotaxic centre (inhibitory, preventing over inflation of the lungs) and the apneustic centre (stimulatory, prolonging inhalation) situated in the pons, higher in the brainstem, adjusts the activity of the respiratory centre (Waugh and Grant, 2018).
Centrally and peripherally located receptors detect changes in arterial partial pressures of oxygen and carbon dioxide. Peripheral chemoreceptors can be found in the carotid bodies and in the arch of the aorta. They send impulses to the respiratory centre to alter ventilation when they detect changes to PO2, PCO2 and H+. The peripheral chemoreceptors are more sensitive to carbon dioxide levels than oxygen levels. Central chemoreceptors are found on the surface of the medulla oblongata, primarily responding to hypercapnia (excessive carbon dioxide levels). These central chemoreceptors are bathed in cerebrospinal fluid, so when there is a rise in PCO2, carbon dioxide crosses the blood–brain barrier from cerebral blood vessels to the cerebrospinal fluid, where hydrogen ions are released. These hydrogen ions are detected by the central chemoreceptors, which send impulses to the respiratory centre to increase the ventilation rate, thereby decreasing the PCO2.
Physiological changes in pregnancy
During pregnancy, there are increased metabolic demands of the maternal and fetal tissues. To compensate for this, there is an increase in metabolic rate and maternal respiratory effort, whereby minute ventilation increases by 15% and approximately 16–20% more oxygen is consumed (Table 1). The expanding uterus also affects the respiratory system as it grows. The elevation of the maternal diaphragm in late pregnancy results in functional residual capacity falling by 20% in the third trimester. However, movement of the diaphragm and therefore vital capacity remain unchanged.
Physiological variable | Change |
---|---|
Oxygen consumption | Increases by ~20% |
Metabolic rate | Increases by ~15% |
Minute ventilation | Increases by ~15% |
Tidal volume | Increases |
Functional residual capacity | Decreases by ~20% in third trimester |
Vital capacity | Unchanged |
PaO2 | Increases |
PaCO2 | Decreases |
Arterial pH | Increases |
The upper respiratory tract and airway mucosa are also affected by hormonal changes in pregnancy. Although airway patency and gaseous exchange across the alveoli remain stable in pregnancy, increasing oestrogen levels cause airway mucosal hyperaemia, oedema, hypersecretion and friability (LoMauro and Aliverti, 2021). Oestrogen also plays a part in increasing the number and sensitivity of progesterone receptors in the hypothalamus and medulla. Increasing progesterone levels in pregnancy increases the sensitivity of the respiratory centre to carbon dioxide levels. Both hormones also increase the hypoxic sensitivity of peripheral chemoreceptors. A relaxation of the bronchioles can also result from increased levels of progesterone, sometimes leading to some dyspnoea. During labour, an increase in tidal volume and respiratory rate may be caused by pain levels. After birth, the changes in the respiratory system rapidly return to normal because of the delivery of the placenta, which leads to a fall in progesterone levels.
Respiratory conditions in pregnancy
Breathlessness or dyspnoea is the sensation of feeling out of breath or the inability to catch your breath. Breathlessness in pregnancy is common (Box 2) and occurs in approximately three-quarters of women at some time during pregnancy but is most common during the third trimester (Nelson-Piercy, 2020). Approximately 50% of women experience breathlessness by 20 weeks and around 70% by 30 weeks of pregnancy, which can potentially cause diagnostic confusion (Nelson-Piercy, 2020). Breathlessness can be the result of many diagnoses, including generalised dyspnoea, asthma, cardiac disease, arrhythmia or pulmonary embolism.
Stages of breathlessness in pregnancy
Based on: Nelson-Piercy (2020)
Asthma
Asthma is a common chronic inflammatory condition of the lung airways and affects approximately 7% of women of childbearing age, occasionally being first diagnosed in pregnancy (Nelson-Piercy, 2020). Asthma is caused by allergen exposure or triggers initiating an inflammatory response, for example, smoking, pollen allergy, exercise, drugs or pollution. Exposure to allergens or triggers cause an overactivity in the inflammatory response of the bronchioles in the lungs. T lymphocytes overproduce cytokines, which stimulate the production and release of antibodies and immunoglobulin E. These antibodies bind to mast cells in the lungs, leading to the release of histamine, prostaglandin and thromboxane A2. These chemicals cause the mucous membrane and muscle layers of the bronchioles to thicken, reducing airflow in the lungs and the muscles go into spasm (bronchospasm). The mucous glands also enlarge and there is excessive secretion of thick, sticky mucus, which exacerbates the airway narrowing. The cytokine increases also causes leukocytes, such as eosinophils and platelets, to accumulate in the airways, which cause epithelial damage (Menzies-Gow et al, 2021).
If asthma is well controlled in pregnancy, there is a low risk of adverse maternal and fetal complications. Therapy should be optimised to reduce the risk of acute asthma attacks. There is more risk to the pregnant woman and her fetus as a result of poorly controlled severe asthma than there is from the medications used to prevent or treat it. Common symptoms of asthma, which are usually worse at night and in the early morning, include shortness of breath, chest tightness, episodes of wheeziness and a cough.
Penumonia
Although there is no increased frequency of pneumonia in the pregnant population compared to the general population, pneumonia in pregnancy can be more virulent with increased mortality (Goodnight and Soper, 2005). Women with a pre-existing history of asthma, cystic fibrosis, anaemia, smoking or an immunosuppressive state increase the risk of contracting pneumonia. Around 40% of antenatal admissions to intensive care units are for pneumonia (Nelson-Piercy, 2020). Common symptoms of pneumonia include:
Tuberculosis
Tuberculosis is a major health concern worldwide in low-income countries where prevention or treatment may not be readily available. Approximately 1.2 million deaths from tuberculosis are estimated to occur worldwide and it is the 13th leading cause of death (Wingfield et al, 2020). Mycobacterium tuberculosis is the main bacteria, causing caseating granulomas in the lungs. Little evidence exist of the effects of pregnancy and tuberculosis, and treatment should not be delayed. Bacillus Calmette-Guérin vaccination should be recommended to all neonates at risk of tuberculosis because of contact in pregnancy or parental origin from a high-risk country. Common symptoms of tuberculosis include cough, haemoptysis, weight loss and night sweats.
Pulmonary embolism
A pulmonary embolism is a thromboembolic event caused by a blockage, usually a blood clot, in the pulmonary artery, potentially preventing blood flow to the lungs. Usually, the cause is from a deep vein thrombosis in the leg or pelvis, which travels through the circulation to the lungs to cause a pulmonary embolism. Damage to blood vessels can cause the formation of a blood clot. Common symptoms of a pulmonary embolism are dyspnoea, tachypnoea, pleuritic pain, cough and haemoptysis.
Cystic fibrosis
Cystic fibrosis is a genetic, autosomal recessive disorder affecting the exocrine glands, leading to an increase in mucus secretions and ion transport, particularly sodium and chloride, at the glands' epithelial cells. It results when the cystic fibrosis transmembrane conductance regulator protein is either not made or is made incorrectly. The respiratory, gastrointestinal, endocrine and reproductive systems are all affected by this disorder. It affects 1 in 25 Caucasian people (Walters and Mehta, 2007), with much lower reported incidence in other ethnic groups, but limitations in gathering accurate data (World Health Organization, 2004). Common symptoms are:
A multidisciplinary approach is key when planning the care of a woman with cystic fibrosis. Risks to the fetus include preterm birth, intrauterine growth restriction and cystic fibrosis diagnosis (Amar et al, 2024).
Smoking
It has long been established that smoking is linked to maternal and infant mortality and morbidity (Tarasi et al, 2022). Maternal smoking has been shown to decrease the amount of oxygen transported to the fetus in utero. Carbon monoxide from cigarette smoke binds with haemoglobin, instead of oxygen, to make carboxyhaemoglobin, reducing the number of binding sites on haemoglobin molecules for oxygen (Tarigan, 2021). Studies have linked maternal smoking in pregnancy and the postnatal period to adverse neonatal outcomes, including low birth weight, premature birth and sudden infant death syndrome (Avşar et al, 2021).
Conclusions
Midwives require a working knowledge of normal physiology and the most common pathophysiology and diseases to provide individualised care to women. They need to be aware of the ways in which the risk of serious morbidity and mortality during pregnancy from respiratory pathology can be reduced. Pregnancy, labour and the postnatal period increase demand on the respiratory system because of the increased metabolic demands of the mother and fetus.
There are many diseases that affect the respiratory system and pregnancy is an added risk factor if a woman has any pre-existing respiratory condition or develops a respiratory disease during pregnancy or the postnatal period. Treatment and medication for respiratory conditions should always follow policy and protocol for any contraindications in pregnancy. A holistic and multidisciplinary approach, considering the woman's wishes, providing psychosocial support and clearly outlining the risks and care plan will result in the best outcomes for women and babies..